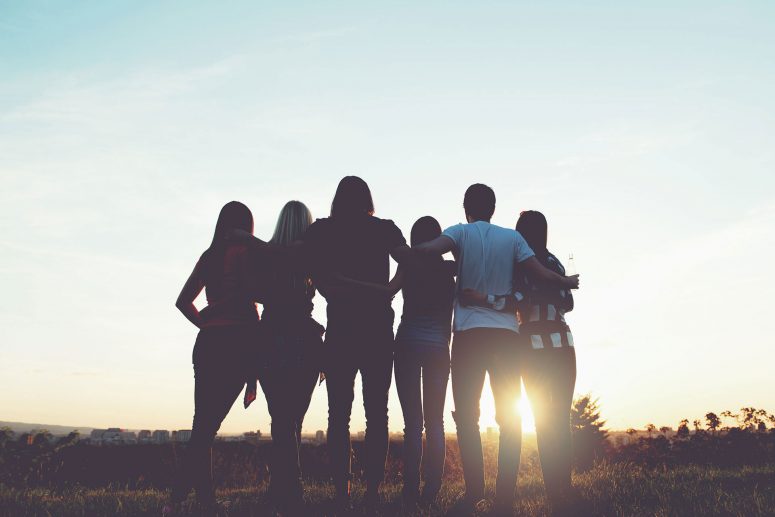
LI_ION battery life cycle aspects
Posted on February 28, 2022
Lifetime Analyses of Lithium-Ion EV Batteries
With global consensus emerging on the paths to a low-carbon sustainable future, the world is looking towards rapid electrification of mobility solutions. The current eneration of Electric Vehicles (EVs) employ Lithium-Ion batteries as the preferred energy storage and delivery technology. It is known that LithiumIon batteries degrade in performance during their service life. This is a matter of concern for consumers, as the Battery pack is significant percentage of EV cost.
Lithium-ion Battery estimated lifetimes typically stated by EV manufacturers1,2 range from 5 to 10 years. However, consumers would be well-advised to obtain additional information since Lithium-Ion battery lifetimes depend on many factors. This White Paper analyses Lithium-Ion EV battery degradation in terms of underlying causes and ey battery performance parameters.
Key takeaways:
• In the global mobility transition to Low Carbon solutions, EVs powered by Lithium-Ion batteries play an important role.
• At present manufacturers indicate battery lifetimes from 5 to 10 years.
• The most common commercial Lithium-Ion battery technologies for EV applications at present are NMC-C and NCA-C.
• Aging of Lithium-Ion EV batteries is a continuous and complex process, dependent on electrochemical phenomena and operational parameters.
• Calendar aging which happens during EV inactivity, aging by driving and charge-discharge cycles are the three distinct operational modes in which battery degradation an be analysed.
• Structural changes to the cathode lattice, SEI layer deposition on Anodes and Lithium plating
of Anodes are major electrochemical phenomena involved in battery degradation.
• High charging rates, high discharge rates, extreme temperature all contribute to battery degradation
Overview of Lithium-Ion Batteries
Before delving into lifetime analyses, an overview of Lithium-ion battery working principles and technology will facilitate the discussion. Lithium-ion batteries are a family of rechargeable batteries utilizing negative and positive electrodes (Anode and Cathode respectively) immersed in a Lithium containing electrolyte that permits migration of Lithium ions.
3 Electrical charge transfer occurs within these batteries by movement of positively charged Lithium ions through the electrolyte, driven by Electrode potential ifference. During discharge of a cell, Lithium ions move from the Anode to the Cathode. The direction
of Lithium ion movement is reversed when the battery is being charged. 4 A variety of Anode and Cathode materials have been used and all of them allow Lithium ions to be parked inside in their lattice structures (termed ‘intercalation’). Thus, the discharge and charge cycles of a Lithium-ion battery involve shuttling of Lithium ions between their parking slots at the Anode and Cathode. While the Anode, Cathode and Electrolyte are responsible for all electrochemical phenomena that power the cell, here are other important structural components 3,5 that play various roles in the functioning of a Lithium-Ion Cell. These are:
Current Collector: These are components attached to each electrode that perform electrical charge collection and transfer between the Lithium-ion cell and the external
circuit. They also act as physical supports for Anode and Cathode materials. At present, commercial Lithium-Ion cells use Copper and Aluminium foils as Collectors at nodes and Cathodes respectively, though other materials such as Nickel, Titanium, Stainless Steels and Carbonaceous materials and structures are being developed.
Separator: The purpose is to prevent accidental short-circuiting of the cell. It is typically a porous polymer membrane that allows Lithium ion movement, while acting as a physical barrier that prevents the Anode and Cathode from touching each other.
Can and Casing: These are needed to avoid ingress of moisture and oxygen and to maintain mechanical integrity. Casing materials used include Aluminium, Nickel coated steels and polymers like Polypropylene.
A EV battery pack would comprise hundreds of connected individual Lithium-Ion battery cells. An electronic Battery Management System (BMS) controls the charge and discharge processes. It also protects the complete system from operating outside the safe range. There would be auxiliary systems to cool the battery and to absorb energy from regenerative braking.
Currently Six types of Lithium-ion Technologies are in commercial use6, as summarized in Table 1. It is apparent that the preferred technology varies for different applications.
Anode material | Cathode material | Remarks |
Carbon (Graphite) | Lithium Cobalt Oxide (LCO) | Used only for consumer electronics, not suitable for EV applications |
Carbon (Graphite) | Lithium Nickel Manganese Cobalt Oxide (NMC) | The cathode combination ratio is usually one-third Nickel, one- third Manganese and one-third Cobalt (NMC-111), but other proportions are also being developed (e.g., NMC -811). NMC’s have a high number of charge and discharge cycles (about 5000 cycles). This battery is most common in power tools and in powertrains for vehicles. |
Carbon (Graphite) | NCA (combination of Oxides of Lithium, Nickel, Cobalt with Aluminium added for stability) | NCA batteries are used for EVs and high-energy applications such as backup power by utility companies. These batteries have about 60-70 cycles (charge and discharge) per year and a total of about 1500 cycles. Typically, Lithium-Ion NCA batteries use a combination of 80% Nickel, 15% Cobalt and 5% Aluminium |
Carbon (Graphite) | LFP (Lithium Iron Phosphate) | Used to be popular for EVs but now being phased out in favour of NMC/NCA types and emerging technologies including Sodium Ion type |
LTO (Lithium Titanium Oxide) | NMC | Not used for EVs, poor specific energy and high costs. But scores high on safety. |
LTO | LFP | Like NMC-LTO, not suitable for EVs |
The electrode combinations that are being used in the present generation of commercial EV Lithium-ion battery packs are either NMC-C or
NCA-C. Hence the focus of this White Paper is on these two technologies.
Key Performance Indicators of Lithium-Ion batteries
How do we measure Lithium-Ion battery capabilities and performance? What are the parameters to be monitored during the useful lifetime of a battery? EV manufacturers tend to highlight vehicular features such as range, speed and power, but not much about the
battery pack that delivers the motive power. The average consumer therefore has a need to research battery related aspects, to make informed purchase decisions. Key performance parameters and values are provided in Table 2 for the NMC-C and NCA-C types.
PARAMETER | SIGNIFICANCE | EV BATTERY TYPE | |
NMC-C | NCA-C | ||
Nominal Cell Capacity (Ah) | This is the total Ampere-hours available when the cell discharges at a specified discharge current (C-rate) from a full charge to the minimum allowable voltage (cut-off voltage). Multiply the discharge Amperes by time of discharge in hours to get Ampere-hours | 25 to 59 | 3.2 |
Nominal Cell Voltage (V)i | The reported voltage of the battery. The actual voltage of a cell varies during discharge. | 3.7 | 3.6 |
Maximum charging current | Lithium-ion battery charging happens in two stages, a constant current stage in which the charge current is controlled and then at constant voltage, when the maximum voltage is reached (very low current) | 1C | 0.7C |
Maximum discharge current | The maximum current at which the battery can be discharged continuously till cut-off voltage | 2C | 1C |
Acceptable Temperature (0C) | The normal acceptable temperature range in which the battery can operate | 0 to 45 | 0 to 45 |
Gravimetric Energy Density (wh/kg) | The nominal battery energy per unit mass, reflects the battery weight required to achieve a given electric range | 130 to 241 | 236 |
Volumetric Energy Density (wh/L) | The nominal battery energy per unit volume, reflects battery size required to achieve a given electric range. | 215 to 466 | 673 |
Battery pack capacity (kwh) | Several thousands of individual cells must be connected together to form a battery pack for an EV | 17 to 60 | 60 to 100 |
Range (km) | This is the maximum range per recharge for an EV | 145 to 400 | 330 to 500 |
Cycle Life (related to depth of discharge and temperature) | The number of discharge-charge cycles the battery can experience before it fails to meet specific performance criteria. Cycle life is estimated for specific charge and discharge conditions | 1000-2000 | 500 |
Thermal runaway | An uncontrollable exothermic reaction when lithium-ion batteries are short (e.g., if separator fails). | 210°C (410°F) typical. | 150°C (302°F) typical, |
Cost | Unit rate per kwh, to be multiplied by capacity | ~$420 per kWh | ~$350 per kWh |
Additionally, the following terms are often used when discussing Lithium-Ion battery performance:
State of Charge (SoC): An expression of the present battery capacity as a percentage of maximum capacity. SoC is generally calculated using current integration to determine the change in battery capacity over time.
Depth of Discharge (DoD): The percentage of battery capacity that has been discharged expressed as a percentage of maximum
capacity. A discharge to at least 80 % DoD is referred to as a deep discharge.
C-Rate and E-Rate: Discharge current is often expressed as a C-rate so that different battery capacities can be compared on a normalized basis.
A C-rate is a measure of the rate at which a battery is discharged relative to its maximum capacity. A 1C rate means that the discharge current will discharge the entire battery in 1 hour. A 1E rate is the discharge power to discharge the entire battery in 1 hour.
Why do Lithium Ion EV Batteries Age?
Aging of batteries during service life is a continuous process. When a battery ages, the immediately observable effects are a loss of capacity and power capability. The range of the EV is also reduced. While there is a vast amount of research literature on Lithium-Ion Battery aging, the following findings are recognized for current
NMC/NCA EV battery technologies:
The lifetime performance of a Lithium-Ion battery is a complex function of numerous physico-chemical processes, influenced by the way the battery system is operated.
The types of aging can be broadly classified as 8:
• Calendar aging: This occurs during inactive periods, when the EV is parked. Temperatures above 25 °C accelerate calendar aging rate, which doubles 40 °C. The SoC value also influences the calendar aging rate in a non-linear fashion.
• Aging by EV Driving Operation: The temperature variations and repeated charge and discharge cycles, as well as patterns of use, result in battery degradation.
• Aging by Charging: High charging rate and low temperatures during charging can degrade the Battery, primarily due to Lithium plating.
Degradation of Lithium-Ion batteries generally follows a linear pattern, with accelerated capacity fade at the beginning and end of each cycle. On this basis, three phases of capacity fade have been identified 9:
• Phase 1: Solid Electrolyte Interphase layer (SEI) coats the anode causing a sudden drop in capacity. SEI is formed from Electrolyte decomposition products of electrolyte solvent and Lithium salt, a reaction that is accelerated by higher temperatures.
• Phase 2: Linear degradation, linked to loss of Lithium that is consumed in various side reactions.
• Phase 3: Rapid capacity fade toward end of life, caused by battery internal resistance increasing.
The main physico-chemical phenomena that damage the battery components and cause aging are8:
• Irreversible structural changes in the cathode active material.
• Loss of particle-to-particle or particle-to-collector bonds as a result from intercalation induced volumetric changes in the active materials.
• Lithium plating: This is the deposition of metallic Lithium onto the Carbon (graphite) Anode. It is accentuated by high charging rates and low temperatures.
The Aging process is influenced by cycling variables, depth of discharge and discharge rate, but this varies depending on battery chemistry.
Cycling variables: The SoC, DoD, C-Rate, E- rate, number of cycles, related temperatures and voltages are all cycling variables. The pattern of EV driving influences the E-rate and extent of discharge (DoD) while charging rates and desired SoC determine the duration of the charge cycle. For example, it has been reported8 that in some European locations, the average EV driving duration is about 4-5% of a day and the daily cycle depth is in the range from 6% to 31%. An average EV use scenario in such cases could be 20 h per day of battery inactivity, 1 h of driving operation and 3 h of charging. Obviously in such cases, Calendar aging mechanisms would predominate. On the other hand, if the EV is used for long distance travel, the cycling variables would be different. The load carried by the EV would also have an influence on the cycling variables. In such cases, EV driving and charging operations would play a greater role in the battery aging processes. Use of Car heaters and Airconditioning which becomes necessary in extreme climates, will affect the battery
discharge rate. The C-rate, E-rate and voltage to which the battery can damage the battery if they exceed the recommended range.11 It is better to avoid the use of Fast charging stations12 since they can adversely affect the battery lifetime. One of the reasons is that the temperature increases rapidly, which accelerates electrolyte decomposition13 and other electrochemical reactions.
Depth of Discharge: Deep Discharge protection is provided by the Battery Management System. If the discharge voltage is lower than 2.7V, it will lead to permanent damage. There is an inverse relationship between current and discharge depth, with current deceasing as DoD increases. At deep discharge, it is observed that voltage and current become unstable and repeating this frequently damages the battery.
14 Discharge rate: Whenever the EV must deliver extra power, the discharge rate increases. Driving uphill, carrying a load, sudden acceleration are all situations that cause the EV battery to increase discharge rate. Excessive current causes temperature rise inside the lithium-ion battery, leading to damage.14
1 Electric Car Battery Life, Cost of Replacement, Recycling & Leasing | EDF (edfenergy.com)
2 How Long Do Electric Car Batteries Last? – TrueCar Blog
3 Lithum-ion Battery (lithiumionbattery.org)
4How lithium-ion batteries works? | SCiB™ Rechargeable battery | Toshiba (global.toshiba)
5Electrochem | Free Full-Text | Review of the Design of Current Collectors for Improving the Battery Performance in Lithium-Ion and Post-Lithium-Ion Batteries (mdpi.com)
6 BU-205: Types of Lithium-ion – Battery University
7 Automotive Li-Ion Batteries: Current Status and Future Perspectives (Journal Article) | DOE PAGES (osti.gov)
8 (PDF) Lifetime Analyses of Lithium-Ion EV Batteries (researchgate.net)
9 Degradation of Commercial Lithium-Ion Cells as a Function of Chemistry and Cycling Conditions – IOPscience
10 ResearchGate (Cheminform Abstracts: Fundamental Degradation Mechanisms of Layered Oxide Li-Ion Battery Cathode Materials: Methodology, Insights and Novel Approaches)
11 Battery Lifetime: How Long Can Electric Vehicle Batteries Last? – CleanTechnica
12 How Long Should An Electric Car’s Battery Last? (myev.com)
13 Why do lithium-ion batteries degrade over time? – AirQualityNews 14The depth of discharge of lithium-ion battery – Why is it necessary to learn (maxworldpower.com)